Unravel the mysteries of the quantum universe through the groundbreaking Copenhagen interpretation. In the early 20th century, a group of pioneering physicists including Niels Bohr and Werner Heisenberg developed a revolutionary framework for understanding the bizarre behavior of particles at the quantum scale.
The Copenhagen interpretation grappled with the fundamental limits of measurement and observation. It proposed that a quantum system exists in a superposition of all possible states until measured or observed, at which point it collapses into a definite state. This counterintuitive idea challenged classical notions of objective reality.
The implications were profound and unsettling. Heisenberg’s uncertainty principle revealed the impossibility of simultaneously measuring certain pairs of properties, like position and momentum, with perfect precision. The more you know about one, the less you can know about the other. This inherent uncertainty is not a limitation of measurement, but a fundamental feature of nature itself.
Einstein famously clashed with Bohr over the completeness of quantum mechanics, arguing that “God does not play dice.” But the Copenhagen view prevailed, backed by experimental evidence. Today, quantum principles power revolutionary technologies like cryptography, computing, and sensing.
Yet the debate still simmers. Alternative interpretations seek to resolve quantum paradoxes, while researchers push the boundaries, pursuing phenomena like entanglement and quantum advantage. Nearly a century later, the Copenhagen legacy remains central to our quest to comprehend reality at its deepest level. As we stand on the brink of a quantum future, grasping its foundations has never been more vital.
The Birth of Quantum Physics
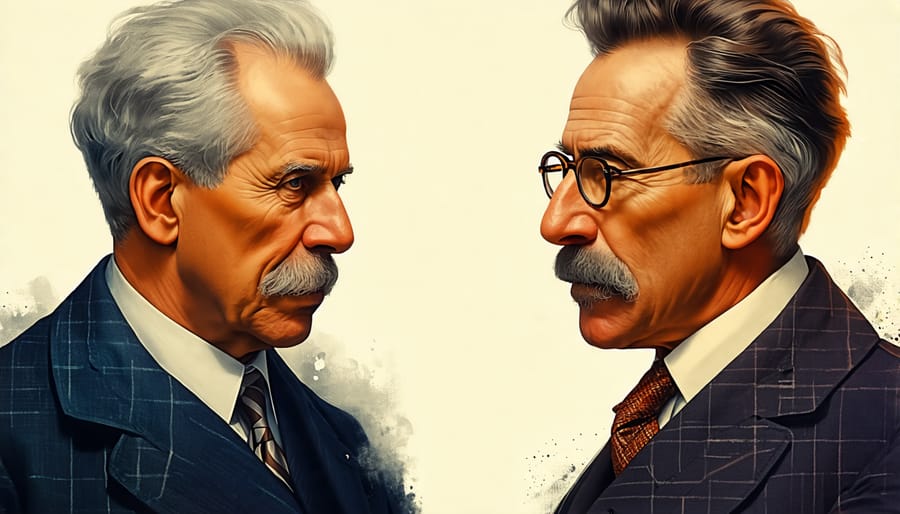
Planck’s Quantum Theory
In 1900, German physicist Max Planck introduced a revolutionary concept that would lay the foundation for quantum physics. Planck proposed that energy is emitted or absorbed in discrete packets called “quanta,” challenging the prevailing belief in the continuous nature of energy. Initially met with skepticism, Planck’s quantum hypothesis successfully explained the puzzling behavior of black-body radiation. As physicist James Clerk Maxwell noted, “The idea of a quantum of energy is startling, but it seems to remove some of the difficulties which beset the theory of radiation.” Planck’s groundbreaking work paved the way for further developments in quantum theory, including Einstein’s explanation of the photoelectric effect and Bohr’s model of the atom. Despite its initial reception, the quantum concept would become a cornerstone of modern physics, leading to a profound shift in our understanding of the subatomic world and its far-reaching implications for technology and our daily lives.
Einstein and the Photoelectric Effect
Albert Einstein played a pivotal role in the development of quantum theory, particularly through his groundbreaking work on the photoelectric effect. In 1905, Einstein proposed that light consists of discrete packets of energy called photons, challenging the prevailing wave theory of light. He suggested that when light strikes a metal surface, it can eject electrons from the metal, but only if the photons have sufficient energy. This explanation of the photoelectric effect revolutionized our understanding of the interaction between light and matter, earning Einstein the Nobel Prize in Physics in 1921. Einstein’s work laid the foundation for the quantum mechanics developed in Copenhagen, even though he later expressed reservations about the probabilistic nature of the theory. As Niels Bohr, a central figure in the Copenhagen interpretation, once said, “Einstein, in his later years, said that the idea of a personal God was childish and he didn’t believe in a personal God. He said he believed in Spinoza’s God who reveals himself in the orderly harmony of what exists.” Einstein’s contributions to quantum theory, including his work on the photoelectric effect, were instrumental in shaping the scientific discussions that took place in Copenhagen and beyond.
Key Principles of the Copenhagen Interpretation
Wave-Particle Duality
One of the most profound insights from the Copenhagen interpretation is the concept of wave-particle duality. This principle states that quantum entities, such as electrons and photons, exhibit both wave-like and particle-like properties depending on the experimental setup. As Niels Bohr, one of the architects of the Copenhagen interpretation, put it, “The opposite of a correct statement is a false statement. But the opposite of a profound truth may well be another profound truth.”
In the famous double-slit experiment, a single electron can pass through two slits simultaneously, creating an interference pattern characteristic of waves. However, when the electron is detected, it behaves as a localized particle. This duality challenges our classical intuition and suggests that the nature of reality at the quantum scale is fundamentally different from our everyday experience.
The Copenhagen interpretation asserts that the wave function, which mathematically describes the quantum state of a system, is not a physical entity but rather a tool for predicting the probabilities of measurement outcomes. The act of measurement itself causes the wave function to “collapse,” randomly selecting one of the possible outcomes according to the probabilities encoded in the wave function. This collapse is known as the “measurement problem” and remains a topic of ongoing research and philosophical debate.
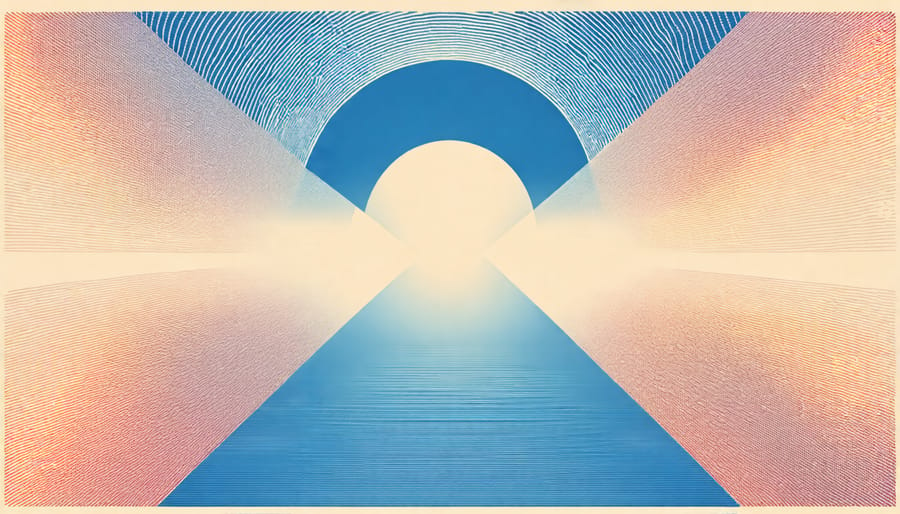
The Uncertainty Principle
Heisenberg’s uncertainty principle, a cornerstone of quantum physics, states that the more precisely we know a particle’s position, the less precisely we can know its momentum, and vice versa. This principle has profound implications for measurement in the quantum realm. As Niels Bohr noted, “It is wrong to think that the task of physics is to find out how nature is. Physics concerns what we can say about nature.”
When we measure a quantum system, we inevitably disturb it, altering the very properties we sought to measure. This inherent limitation means that we can never have a complete picture of a quantum system at any given moment. The uncertainty principle also gives rise to fascinating phenomena like the wave-particle duality, where quantum entities exhibit both wave-like and particle-like properties depending on how they are measured. As physicist Werner Heisenberg remarked, “We have to remember that what we observe is not nature herself, but nature exposed to our method of questioning.”
The Role of Measurement
The Copenhagen interpretation emphasizes the crucial role of measurement in quantum physics. According to this view, a quantum system exists in a superposition of states until it is measured, at which point the wave function collapses into a definite state. Niels Bohr argued that the act of measurement itself determines the outcome, and that the properties of quantum entities are not inherently defined before observation. This implies that the observer plays an active role in shaping reality at the quantum level. The Copenhagen interpretation’s stance on measurement has been a subject of much debate and philosophical discussion, with some arguing that it introduces a subjective element into the otherwise objective realm of physics. Despite the controversies, the Copenhagen interpretation’s emphasis on the importance of measurement has had a profound impact on our understanding of the quantum world and continues to shape the way we think about the nature of reality.
Implications and Controversies
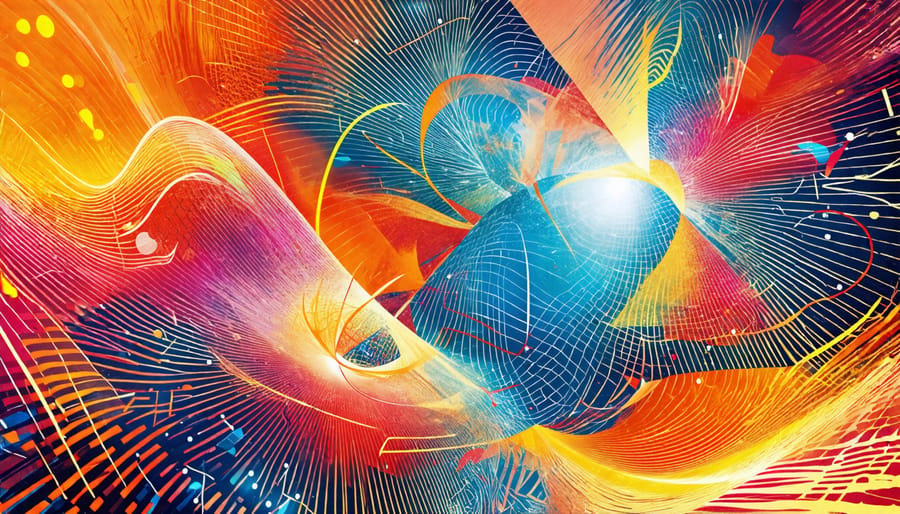
Philosophical Implications
The Copenhagen interpretation of quantum physics has profound philosophical implications that challenge our understanding of reality and the role of the observer. One of the most striking aspects is the notion that particles exist in a state of superposition, simultaneously occupying multiple states until observed. This suggests that reality is not fixed but rather dependent on measurement, blurring the line between the objective and subjective.
The act of observation itself becomes a crucial part of the quantum system, with the observer’s choice of measurement apparatus determining the outcome. This raises questions about the nature of free will and the extent to which we shape our own reality. The Copenhagen interpretation also introduces the concept of complementarity, which posits that certain properties, such as position and momentum, cannot be simultaneously known with precision. This challenges classical notions of causality and determinism.
Furthermore, the interpretation’s emphasis on probabilistic outcomes rather than definite trajectories has led some to question the existence of an underlying reality independent of observation. This has sparked debates about the nature of knowledge and the limits of scientific inquiry. While the Copenhagen interpretation remains the most widely accepted view among physicists, its philosophical implications continue to be a subject of lively discussion and exploration.
Alternative Interpretations
While the Copenhagen interpretation remains the most widely accepted framework for understanding quantum mechanics, several alternative interpretations have emerged over the years. These interpretations seek to address some of the philosophical and conceptual challenges posed by the Copenhagen view, such as the role of the observer and the nature of reality at the quantum scale.
One notable alternative is the many-worlds interpretation, proposed by Hugh Everett in 1957. This interpretation suggests that every quantum event creates a branching of parallel universes, each representing a different outcome. In this view, the wave function never collapses, and all possible outcomes of a quantum measurement occur in different universes.
Another prominent alternative is the de Broglie-Bohm theory, also known as the pilot wave theory. This interpretation posits that particles have well-defined positions and velocities, guided by a quantum potential or “pilot wave.” This approach attempts to reconcile quantum mechanics with a more deterministic worldview, although it still maintains the probabilistic nature of quantum measurements.
Other interpretations, such as the consistent histories approach and the quantum Bayesianism (QBism) interpretation, have also gained traction in recent years. These alternatives aim to provide a more coherent and intuitive understanding of quantum phenomena, which could potentially guide the development of next-generation quantum computers and other quantum technologies.
As quantum physics continues to evolve, the debate surrounding these interpretations remains active and vital to the progression of the field.
Conclusion
The Copenhagen interpretation of quantum physics has had a profound and lasting impact on our understanding of the fundamental nature of reality. Its key principles, such as the wave-particle duality, the uncertainty principle, and the role of measurement in determining quantum outcomes, have revolutionized our view of the subatomic world. These ideas challenged classical notions of determinism and causality, revealing a universe governed by probability and inherent uncertainties.
The interpretation’s emphasis on the observer’s role in measuring quantum systems has sparked philosophical debates about the nature of reality and the relationship between the observer and the observed. It has led to thought experiments like Schrödinger’s cat, which highlight the peculiarities of quantum superposition and the collapse of the wave function upon measurement.
Despite its counterintuitive implications, the Copenhagen interpretation has been remarkably successful in predicting and explaining a wide range of quantum phenomena. It has laid the foundation for advances in fields such as quantum computing, cryptography, and sensing, which hold immense potential for transformative technologies and quantum breakthroughs.
However, the interpretation is not without its critics or alternative theories. Some researchers argue that it leaves fundamental questions unanswered, such as the nature of measurement and the origin of quantum randomness. Competing interpretations, like the many-worlds interpretation and hidden variable theories, offer different perspectives on these issues.
Nonetheless, the Copenhagen interpretation remains a cornerstone of quantum physics, shaping our understanding of the subatomic realm and inspiring new avenues of research. As we continue to explore the frontiers of quantum science, the interpretation’s insights and challenges will undoubtedly continue to drive scientific progress and deepen our appreciation of the strange and wonderful world revealed by quantum physics.